功率放大器的分类与原理
发布日期:2023-05-11
点击次数:1037
功率放大器的分类
甲类工作状态:整个工作周期内晶体管的集电极电流始终是流通的,放大器的效率最低,带来的是非线性失真度比较小。一般用于对失真比较敏感的场合,比如HI-FI音响。
乙类工作状态:半个周期工作另半个周期截止,乙类工作状态也称为B类工作状态。两只互补的晶体管推挽工作,效率比甲类功放高,但存在交越失真的问题,一般功率放大器采用这种形式。
甲乙类工作状态:它是介于甲类和乙类之间的工作状态,即晶体管工作周期大于一半,这种功放的特性介于甲类和乙类。
丙类工作状态:这种状态下,晶体管工作的时间小于半个周期,丙类工作状态又称为C类工作状态,丙类功放一般用于高频的谐振功放。
丁类工作状态:把声音信号调制为PWM形式,晶体管工作在开关状态,输出端通过LC滤波器恢复信号波形。效率高,高频特性差,用于小型化电池供电以及要求高效率的场合。
根据工作状态的不同,功率放大器分类如下:
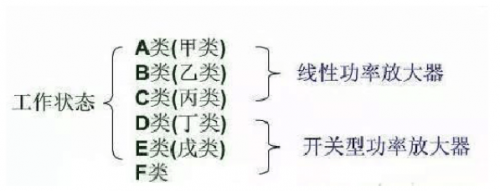
传统线性功率放大器的工作频率很高,但相对频带较窄,射频功率放大器一般都采用选频网络作为负载回路。射频功率放大器可以按照电流导通角的不同,分为甲 (A)、乙(B)、丙(C)三类工作状态。甲类放大器电流的导通角为360°,适用于小信号低功率放大,乙类放大器电流的导通角等于180°,丙类放大器电流的导通角则小于180°。乙类和丙类都适用于大功率工作状态,丙类工作状态的输出功率和效率是三种工作状态中最高的。射频功率放大器大多工作于丙类,但丙类放大器的电流波形失真太大,只能用于采用调谐回路作为负载谐振功率放大。由于调谐回路具有滤波能力,回路电流与电压仍然接近于正弦波形,失真很小。
开关型功率放大器(Switching Mode PA,SMPA),使电子器件工作于开关状态,常见的有丁(D)类放大器和戊(E)类放大器,丁类放大器的效率高于丙类放大器。SMPA将有源晶体管驱动为开关模式,晶体管的工作状态要么是开,要么是关,其电压和电流的时域波形不存在交叠现象,所以是直流功耗为零,理想的效率能达到100%。
传统线性功率放大器具有较高的增益和线性度但效率低,而开关型功率放大器具有很高的效率和高输出功率,但线性度差。具体见下表:
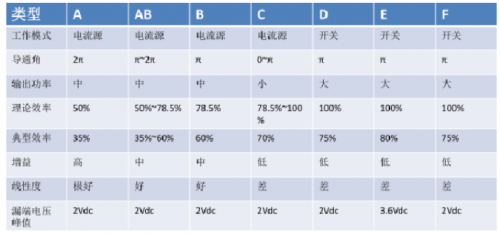
电路组成
放大器有不同类型,简化之,放大器的电路可以由以下几个部分组成:晶体管、偏置及稳定电路、输入输出匹配电路。
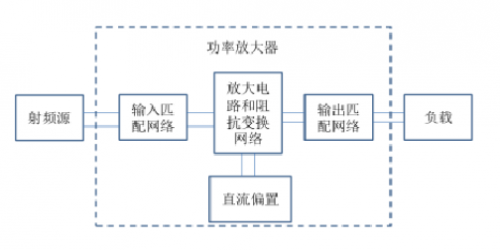
1、晶体管
晶体管有很多种,包括当前还有多种结构的晶体管被发明出来。本质上,晶体管的工作都是表现为一个受控的电流源或电压源,其工作机制是将不含内容的直流的能量转化为“有用的”输出。直流能量乃是从外界获得,晶体管加以消耗,并转化成有用的成分。不同的晶体管不同的“能力”,比如其承受功率的能力有区别,这也是因为其能获取的直流能量的能力不同所致;比如其反应速度不同,这决定它能工作在多宽多高的频带上;比如其面向输入、输出端的阻抗不同,及对外的反应能力不同,这决定了给它匹配的难易程度。
2、偏置电路及稳定电路
偏置和稳定电路是两种不同的电路,但因为他们往往很难区分,且设计目标趋同,所以可以放在一起讨论。
晶体管的工作需要在一定的偏置条件下,我们称之为静态工作点。这是晶体管立足的根本,是它自身的“定位”。每个晶体管都给自己进行了一定的定位,其定位不同将决定了它自身的工作模式,在不同的定位上也存在着不同的性能表现。有些定位点上起伏较小,适合于小信号工作;有些定位点上起伏较大,适合于大功率输出;有些定位点上索取较少,释放纯粹,适合于低噪声工作;有些定位点,晶体管总是在饱和和截至之间徘徊,处于开关状态。一个恰当的偏置点,是正常工作的础。在设计宽带功率放大器时,或工作频率较高时,偏置电路对电路性能影响较大,此时应把偏置电路作为匹配电路的一部分考虑。
偏置网络有两大类型,无源网络和有源网络。无源网络(即自偏置网络)通常由电阻网络组成,为晶体管提供合适的工作电压和电流。它的主要缺陷是对晶体管的参数变化十分敏感,并且温度稳定性较差。有源偏置网络能改善静态工作点的稳定性,还能提高良好的温度稳定性,但它也存在一些问题,如增加了电路尺寸、增加了电路排版的难度以及增加了功率消耗。 稳定电路一定要在匹配电路之前,因为晶体管需要将稳定电路作为自身的一部分存在,再与外界接触。在外界看来,加上稳定电路的晶体管,是一个“全新的”晶体管。它做出一定的“牺牲”,获得了稳定性。稳定电路的机制能够保证晶体管顺利而稳定的运转。
3、输入输出匹配电路
匹配电路的目的是在选择一种接受的方式。对于那些想提供更大增益的晶体管来说,其途径是全盘的接受和输出。这意味着通过匹配电路这一个接口,不同的晶体管之间沟通更加顺畅,对于不同种的放大器类型来说,匹配电路并不是只有“全盘接受”一种设计方法。一些直流小、根基浅的小型管,更愿意在接受的时候做一定的阻挡,来获取更好的噪声性能,然而不能阻挡过了头,否则会影响其贡献。而对于一些巨型功率管,则需要在输出时谨小慎微,因为他们更不稳定,同时,一定的保留有助于他们发挥出更多的“不扭曲的”能量。
典型的阻抗匹配网络有L匹配、π形匹配和T形匹配。其中L匹配,其特点就是结构简单且只有两个自由度L和C。一旦确定了阻抗变换比率和谐振频率,网络的Q值(带宽)也就确定了。π形匹配网络的一个优点就是不管什么样的寄生电容,只要连接到它,都可以被吸到网络中,这也导致了 π形匹配网络的普遍应用,因为在很多的实际情况中,占支配地位的寄生元件是电容。T形匹配,当电源端和负载端的寄生参数主要呈电感性质时,可用T形匹配来把这些寄生参数吸收入网络。