How to fully extend battery life? You should master these power design elements!
Time:2022-06-12
Views:2105
With the continuous development of remote patient monitor (RPM), more and more functions are included, so that doctors can better understand the health status of patients. These functions put forward higher requirements for the single battery supplying power to the monitor. This paper provides a power supply solution for ECG (electrocardiogram) remote patient monitoring patch, which aims to prolong the battery life and give full play to the monitoring role. This paper also introduces the strategy of accurately estimating the battery life of RPM and the method of extending the battery life before rpm is powered on.
The revolution of the Internet of things (IOT) has led to a paradigm shift in the way medical institutions use technology to provide real-time care for patients. Today, remote patient monitoring is one of the areas in which this new medical device changes the way doctors interact with patients. Smaller integrated circuits and wireless communication make the old equipment decades ago updated and enhanced, so as to improve patient compliance and efficacy. The current remote patient monitoring patch replaces the cumbersome Holter device in the past. The patch contains various sensors that can collect heart rate, temperature and accelerometer data. These patches transfer patient data to the cloud, and both patients and doctors can access the data in real time.
Although these devices help doctors improve their nursing ability, they also pose challenges to power designers, who must balance system performance and battery life requirements. As the second-generation patch uses multimodal sensing to improve the accuracy and effectiveness, these challenges are further increased, and higher requirements are put forward for the power supply.
In this article, we will refer to the ECG RPM patch example shown in Figure 1. The patch continuously monitors the ECG and accelerometer while checking the temperature every 15 minutes. Data via low power Bluetooth ® (ble) transmit once every 2 hours, a total of 12 ble transmissions per day. The patch has three different modes, each of which has a different load mode: standard monitoring, temperature monitoring and transmission mode. In standard monitoring mode, only ECG and accelerometer are monitored. In the temperature monitoring mode, another temperature sensor is also monitored. In transmission mode, ble radio transmits data while simultaneously monitoring ECG and accelerometer data.
Figure 1 Schematic diagram of ECG patch power supply. A 235 MAH CR2032 button lithium battery is used to supply power to the voltage regulator, microcontroller, ECG front end, temperature sensor and accelerometer.
Power challenges
Designing RPMs, such as ECG patches, is a multifaceted challenge for power designers. Designs are often limited by space, and patches with multiple sensors may require multiple power rails. Since RPM patches are usually disposable products, designers usually choose button batteries as a cost-effective power supply. If only button batteries are used to power the patch, the designer must also consider the efficiency of the power subsystem.
A challenge that power designers often overlook is how to extend the shelf life of products. Turn off current and battery self discharge will shorten the life of any system. Therefore, the designer must determine whether the RPM patch can meet the running time requirements after the typical shelf life, and if not, what measures can be taken to maintain the battery life before the patch reaches the end user.
Determine battery run time
In order to accurately determine whether the power solution meets the battery life requirements, the load curve must be determined. The load curve is a simple representation of the system load duty cycle. For the remote patient monitoring patch we use, we will consider the three different operating modes previously introduced: standard monitoring, temperature monitoring and transmission mode.
In order to accurately determine whether the power solution meets the battery life requirements, the load curve must be determined. The load curve is a simple representation of the system load duty cycle. For the remote patient monitoring patch we use, we will consider the three different operating modes previously introduced: standard monitoring, temperature monitoring and transmission mode.
For load curve analysis, the time period of each operating mode in a day is used to determine the duty cycle calculation value. Use formula 1:
The duty cycle of the patch can be obtained for us, as shown in Table 1.
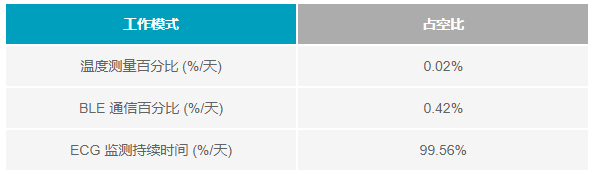
Table 1 Duty cycle of the patch in different operating modes
Using the load curve in Figure 2, we can calculate the current consumption of the patch. Take the effective current consumption under each operating mode, and calculate the approximate value of the average current consumption per day through formula 2.
Figure 2 Load curve.
The following is a calculation example:
Standard monitoring mode current per day = standard monitoring mode current × Standard monitoring mode duty cycle × 24 hours
Standard monitoring mode current = 1.88 Ma standard monitoring mode duty cycle = 0.9956
Current per day in standard monitoring mode = 1.88 Ma × zero point nine nine five six × 24 hours = 44.92 mah/ day
Once the daily current consumption for each operating mode is determined, the service life of the battery can be determined by equation 3.
The following is a calculation example:
Battery capacity = 235 Mah
Current per day in standard monitoring mode = 44.92 mah/ day
Current per day in temperature monitoring mode = 0.01 mah/ day
Current per day in transmission mode = 0.79 mah/ day
Battery life (days) = 235 mah/ (44.92 mah/ day + 0.01 mah/ day + 0.79 ma/ day) = 5.14 days
These results show that the device will meet the working time requirement of 5 days, and the battery life will exceed 5.1 days. However, this result is deceptive because the shelf life of the system is not considered. In the medical device industry, it is better to design a shelf life of 14 months (shelf life of 12 months, transportation period of two months).
Shelf life considerations
Add the off current of the equipment in the system, and use the typical self discharge rate of 1% to 2% of CR2032 battery every year. It can be seen that after 14 months, the capacity of the battery is not enough to support the working time of 5 days, so the battery needs to be sealed.
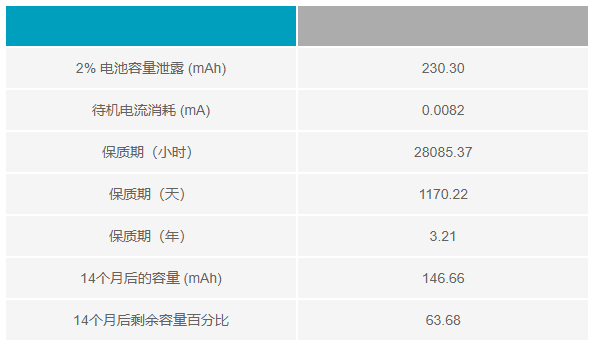
Table 2 Battery capacity after 14 months
After 14 months on the shelf, the battery capacity will be significantly reduced. When CR2032 is idle on the shelf, nearly 40% of the energy will be consumed through turn-off current and battery self leakage. By substituting this battery capacity into formula 3, a more accurate operating time can be obtained:
Battery life (days) = 146.66 mah/ (standard monitoring mode + temperature monitoring mode + transmission mode)
Battery life (days) = 146.66 mah/ (44.92 mah/ day + 0.01 mah/ day + 0.79 ma/ day) = 3.21 days
If the storage time exceeds one year, the battery capacity will be affected by the battery self discharge and the system shutdown current. Battery self discharge is related to the chemical properties and environment of the battery. The chemical composition of CR2032 battery is lithium manganese, and the annual self discharge rate is 1% to 2%. After one year, the button battery may lose 2% of its capacity in the sleep state. In contrast, the chemical composition of br2032 battery is lithium fluorocarbon polymer, and the annual self discharge rate is 0.3%. We usually think that the most suitable battery chemical composition for application is the lowest discharge rate, but this is not the case. Although the discharge rate of br2032 battery is low, its capacity is also lower than that of CR2032 battery of 200 MAH. Using the previous formula to recalculate, you can determine whether such a low-capacity battery has enough power.
In this ECG patch, when the system is powered off, the IC shutdown current is the biggest factor to reduce the battery life. When the IC is disabled and there is no payload, a turn-off current is generated. These currents are usually caused by leakage in the IC and ESD protection devices in the IC. Even without load, a small amount of current will be consumed. These currents are usually small (below 1 μ A) But it has a great impact on battery life. In this RPM chip, the turn off current can reduce the battery capacity by up to 40% in one year. The use of a battery seal limits the system from drawing excessive current from the battery when it is turned off.
There are two ways to seal the battery: mechanical battery seal in the form of polyester film pull tab and electrical battery seal in the form of load switch. The Mylar / plastic tab is a mechanical battery seal in which the plastic tab is located between the battery and the system. When preparing to use the equipment, the user only needs to pull out the plastic sheet, and the battery starts to supply power to the system. This is a simple, low-cost, mature battery mechanical seal, which has been used for many years. However, this solution is not always feasible for medical devices. For ECG patches that need to be waterproof, the protruding groove of polyester film will make the patches vulnerable to water damage. In addition, this small plastic sheet may not be easy to use for the end user who is not smart enough.
Simple load switches, such as Vishay sip32341, are a good choice for electrical battery sealing. The device is a field-effect transistor. When turned on, it can separate the battery from other parts of the system, making the turn-off current of sip32341 the only consumed current on the battery. The load switch has a logic control line, which can be connected through the button when the equipment is ready to be used. The turn-off current of sip32341 is generally 14 Pa. compared with the current consumption of the whole system without battery seal, the use of battery seal has significantly improved. If sip32341 is used as the battery seal, the CR2032 primary battery can maintain 99.97% capacity within 14 months. If the battery seal is not used to protect the battery from the off current of ECG patch, the CR2032 primary battery can only maintain 62.39% of the original power. By eliminating this 37% capacity difference, the ECG patch can still meet the 5-day life requirement after the 14 month shelf life.
Table 3 Battery capacity after 14 months of using the battery seal
The battery seal maintains battery capacity by preventing all devices in the system from consuming battery shutdown current. After the RPM patch was idle for 14 months, the remaining battery capacity remained above 99.9%.
By substituting this battery capacity into formula 3, a more accurate operating time can be obtained:
Battery life (days) = 230.25 mah/ (standard monitoring mode + temperature monitoring mode + transmission mode)
Battery life (days) = 230.25 mah/ (44.92 mah/ day + 0.01 mah/ day + 0.79 ma/ day) = 5.04 days
conclusion
Battery analysis while the system is active and in shutdown / low power mode is critical to designing a power supply that meets all the requirements of medical devices. Although this paper specially discusses the ECG patch that collects heart rate, temperature and acceleration data through ble communication, the analysis and principle in this paper can also be applied to any number of medical equipment systems powered by primary batteries.
The revolution of the Internet of things (IOT) has led to a paradigm shift in the way medical institutions use technology to provide real-time care for patients. Today, remote patient monitoring is one of the areas in which this new medical device changes the way doctors interact with patients. Smaller integrated circuits and wireless communication make the old equipment decades ago updated and enhanced, so as to improve patient compliance and efficacy. The current remote patient monitoring patch replaces the cumbersome Holter device in the past. The patch contains various sensors that can collect heart rate, temperature and accelerometer data. These patches transfer patient data to the cloud, and both patients and doctors can access the data in real time.
Although these devices help doctors improve their nursing ability, they also pose challenges to power designers, who must balance system performance and battery life requirements. As the second-generation patch uses multimodal sensing to improve the accuracy and effectiveness, these challenges are further increased, and higher requirements are put forward for the power supply.
In this article, we will refer to the ECG RPM patch example shown in Figure 1. The patch continuously monitors the ECG and accelerometer while checking the temperature every 15 minutes. Data via low power Bluetooth ® (ble) transmit once every 2 hours, a total of 12 ble transmissions per day. The patch has three different modes, each of which has a different load mode: standard monitoring, temperature monitoring and transmission mode. In standard monitoring mode, only ECG and accelerometer are monitored. In the temperature monitoring mode, another temperature sensor is also monitored. In transmission mode, ble radio transmits data while simultaneously monitoring ECG and accelerometer data.
Figure 1 Schematic diagram of ECG patch power supply. A 235 MAH CR2032 button lithium battery is used to supply power to the voltage regulator, microcontroller, ECG front end, temperature sensor and accelerometer.
Power challenges
Designing RPMs, such as ECG patches, is a multifaceted challenge for power designers. Designs are often limited by space, and patches with multiple sensors may require multiple power rails. Since RPM patches are usually disposable products, designers usually choose button batteries as a cost-effective power supply. If only button batteries are used to power the patch, the designer must also consider the efficiency of the power subsystem.
A challenge that power designers often overlook is how to extend the shelf life of products. Turn off current and battery self discharge will shorten the life of any system. Therefore, the designer must determine whether the RPM patch can meet the running time requirements after the typical shelf life, and if not, what measures can be taken to maintain the battery life before the patch reaches the end user.
Determine battery run time
In order to accurately determine whether the power solution meets the battery life requirements, the load curve must be determined. The load curve is a simple representation of the system load duty cycle. For the remote patient monitoring patch we use, we will consider the three different operating modes previously introduced: standard monitoring, temperature monitoring and transmission mode.
In order to accurately determine whether the power solution meets the battery life requirements, the load curve must be determined. The load curve is a simple representation of the system load duty cycle. For the remote patient monitoring patch we use, we will consider the three different operating modes previously introduced: standard monitoring, temperature monitoring and transmission mode.
For load curve analysis, the time period of each operating mode in a day is used to determine the duty cycle calculation value. Use formula 1:
The duty cycle of the patch can be obtained for us, as shown in Table 1.
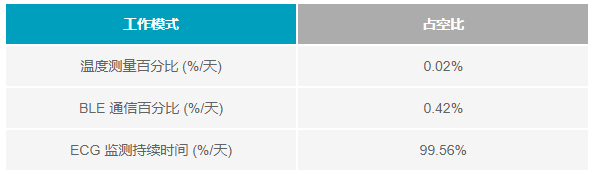
Table 1 Duty cycle of the patch in different operating modes
Using the load curve in Figure 2, we can calculate the current consumption of the patch. Take the effective current consumption under each operating mode, and calculate the approximate value of the average current consumption per day through formula 2.
Figure 2 Load curve.
The following is a calculation example:
Standard monitoring mode current per day = standard monitoring mode current × Standard monitoring mode duty cycle × 24 hours
Standard monitoring mode current = 1.88 Ma standard monitoring mode duty cycle = 0.9956
Current per day in standard monitoring mode = 1.88 Ma × zero point nine nine five six × 24 hours = 44.92 mah/ day
Once the daily current consumption for each operating mode is determined, the service life of the battery can be determined by equation 3.
The following is a calculation example:
Battery capacity = 235 Mah
Current per day in standard monitoring mode = 44.92 mah/ day
Current per day in temperature monitoring mode = 0.01 mah/ day
Current per day in transmission mode = 0.79 mah/ day
Battery life (days) = 235 mah/ (44.92 mah/ day + 0.01 mah/ day + 0.79 ma/ day) = 5.14 days
These results show that the device will meet the working time requirement of 5 days, and the battery life will exceed 5.1 days. However, this result is deceptive because the shelf life of the system is not considered. In the medical device industry, it is better to design a shelf life of 14 months (shelf life of 12 months, transportation period of two months).
Shelf life considerations
Add the off current of the equipment in the system, and use the typical self discharge rate of 1% to 2% of CR2032 battery every year. It can be seen that after 14 months, the capacity of the battery is not enough to support the working time of 5 days, so the battery needs to be sealed.
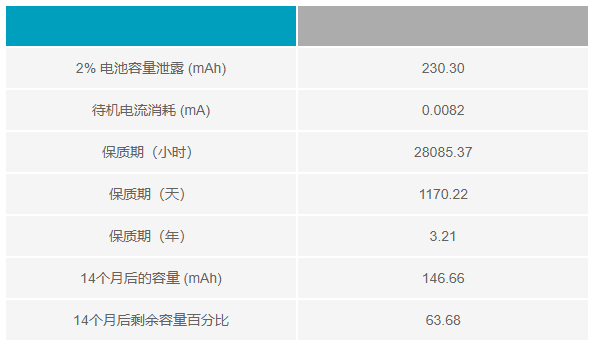
Table 2 Battery capacity after 14 months
After 14 months on the shelf, the battery capacity will be significantly reduced. When CR2032 is idle on the shelf, nearly 40% of the energy will be consumed through turn-off current and battery self leakage. By substituting this battery capacity into formula 3, a more accurate operating time can be obtained:
Battery life (days) = 146.66 mah/ (standard monitoring mode + temperature monitoring mode + transmission mode)
Battery life (days) = 146.66 mah/ (44.92 mah/ day + 0.01 mah/ day + 0.79 ma/ day) = 3.21 days
If the storage time exceeds one year, the battery capacity will be affected by the battery self discharge and the system shutdown current. Battery self discharge is related to the chemical properties and environment of the battery. The chemical composition of CR2032 battery is lithium manganese, and the annual self discharge rate is 1% to 2%. After one year, the button battery may lose 2% of its capacity in the sleep state. In contrast, the chemical composition of br2032 battery is lithium fluorocarbon polymer, and the annual self discharge rate is 0.3%. We usually think that the most suitable battery chemical composition for application is the lowest discharge rate, but this is not the case. Although the discharge rate of br2032 battery is low, its capacity is also lower than that of CR2032 battery of 200 MAH. Using the previous formula to recalculate, you can determine whether such a low-capacity battery has enough power.
In this ECG patch, when the system is powered off, the IC shutdown current is the biggest factor to reduce the battery life. When the IC is disabled and there is no payload, a turn-off current is generated. These currents are usually caused by leakage in the IC and ESD protection devices in the IC. Even without load, a small amount of current will be consumed. These currents are usually small (below 1 μ A) But it has a great impact on battery life. In this RPM chip, the turn off current can reduce the battery capacity by up to 40% in one year. The use of a battery seal limits the system from drawing excessive current from the battery when it is turned off.
There are two ways to seal the battery: mechanical battery seal in the form of polyester film pull tab and electrical battery seal in the form of load switch. The Mylar / plastic tab is a mechanical battery seal in which the plastic tab is located between the battery and the system. When preparing to use the equipment, the user only needs to pull out the plastic sheet, and the battery starts to supply power to the system. This is a simple, low-cost, mature battery mechanical seal, which has been used for many years. However, this solution is not always feasible for medical devices. For ECG patches that need to be waterproof, the protruding groove of polyester film will make the patches vulnerable to water damage. In addition, this small plastic sheet may not be easy to use for the end user who is not smart enough.
Simple load switches, such as Vishay sip32341, are a good choice for electrical battery sealing. The device is a field-effect transistor. When turned on, it can separate the battery from other parts of the system, making the turn-off current of sip32341 the only consumed current on the battery. The load switch has a logic control line, which can be connected through the button when the equipment is ready to be used. The turn-off current of sip32341 is generally 14 Pa. compared with the current consumption of the whole system without battery seal, the use of battery seal has significantly improved. If sip32341 is used as the battery seal, the CR2032 primary battery can maintain 99.97% capacity within 14 months. If the battery seal is not used to protect the battery from the off current of ECG patch, the CR2032 primary battery can only maintain 62.39% of the original power. By eliminating this 37% capacity difference, the ECG patch can still meet the 5-day life requirement after the 14 month shelf life.
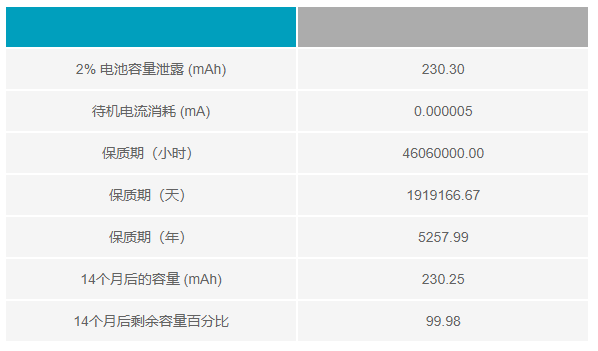
Table 3 Battery capacity after 14 months of using the battery seal
The battery seal maintains battery capacity by preventing all devices in the system from consuming battery shutdown current. After the RPM patch was idle for 14 months, the remaining battery capacity remained above 99.9%.
By substituting this battery capacity into formula 3, a more accurate operating time can be obtained:
Battery life (days) = 230.25 mah/ (standard monitoring mode + temperature monitoring mode + transmission mode)
Battery life (days) = 230.25 mah/ (44.92 mah/ day + 0.01 mah/ day + 0.79 ma/ day) = 5.04 days
conclusion
Battery analysis while the system is active and in shutdown / low power mode is critical to designing a power supply that meets all the requirements of medical devices. Although this paper specially discusses the ECG patch that collects heart rate, temperature and acceleration data through ble communication, the analysis and principle in this paper can also be applied to any number of medical equipment systems powered by primary batteries.
Disclaimer: This article is transferred from other platforms and does not represent the views and positions of this site. If there is infringement or objection, please contact us to delete. thank you! |